Plasmo-electronic
« plasmonic photoconductance in free-standing monolayered gold nanoparticle membranes »
Plasmo-electronic: “plasmonic photoconductance in free-standing monolayered gold nanoparticle membranes”.
In collaboration with Adnen Mlayah (CEMES / CNRS, NeO team) (adnen.mlayah@cemes.fr), we develop the plasmo-electronics field, coupling between plasmonic phenomenon and electron transport of metallic nanoparticles assemblies surrounded by organic ligands.
The Stencil membranes developed in this project are the result of a long collaboration with Jürgen Brugger
(EPFL, https://people.epfl.ch/juergen.brugger)
Plasmonic metal nanostructures have recently attracted extensive research and been exploited for enhancing the performance of various optoelectronic devices [1–4]. Colloids composed of metallic nanoparticles (NPs) coated by organic ligands have been used to fabricate hybrid molecular networks contacted by nanoelectrodes [5–8]. Overall, coupling localized electron oscillations with charge transport leads to an emerging field called “plasmo-electronics” that could provide a new class of light responsive materials. In order to understand the interplay between surface plasmon resonance and electrical conductance and mechanisms at stake, it is primordial to lower the influence of parasite parameters such as substrate of the assemblies and to provide a model system (Fig. 1). So far, we managed to assemble robust, conductive, monolayered, free-standing NP arrays, so called NP membranes.
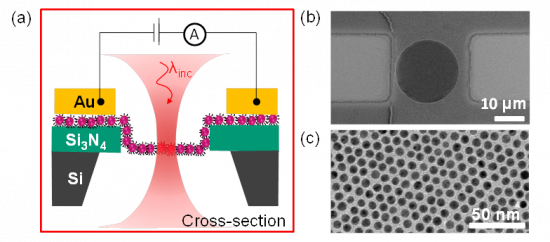
In this work, we investigate the photoconductance generation in NP membranes under focused laser illumination. We carried out that the amplitude of the induced current is correlated with the plasmonic resonance of the NP arrays (Fig. 2a) but also strongly depends of illumination power (Fig. 2b) and polarization voltage. The absence of a substrate also leads to a 50% raise of the photocurrent at the resonant wavelenght. DDA simulations showed that at least half of this increase is due to the optical properties of the substrate. Finally, accordingly to the temperature dependence of electrical conductance, the photocurrent in the free-standing NP membrane can be interpreted as a combination of a trap state dynamics and a thermally activated phenomenon yielding a temperature rise estimated at 40K at plasmon resonance for Ilaser 5.3kW/cm2. Intrinsic plasmo-electronic properties have been measured with no influence of a substrate allowing a superior transduction for better sensing or hyperthermia applications.
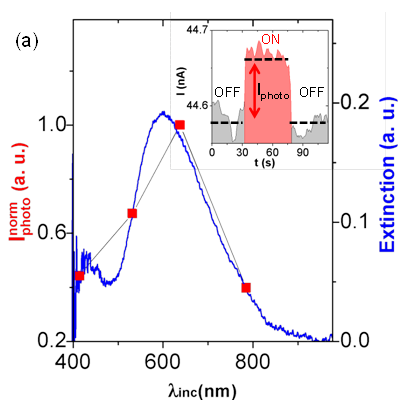
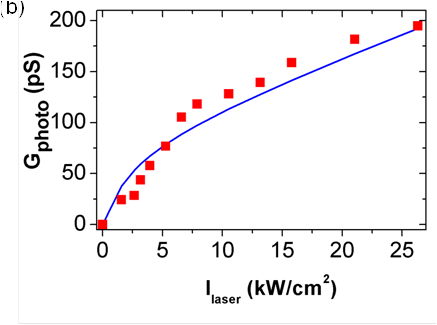
References:
[1] K. R. Catchpole and A. Polman, Appl. Phys. Lett., 2008, 93, 191113.
[2] H. A. Atwater and A. Polman, Nat. Mater., 2010, 9, 205–213.
[3] J. Liao, S. Blok, S. J. van der Molen, S. Diefenbach, A. W. Holleitner, C. Schönenberger, A. Vladyka and M. Calame, Chem Soc Rev, 2015, 44, 999–1014.
[4] T. Hashimoto, Y. Fukunishi, B. Zheng, Y. Uraoka, T. Hosoi, T. Shimura and H. Watanabe, Appl. Phys. Lett., 2013, 102, 083702.
[5] P. Banerjee, D. Conklin, S. Nanayakkara, T.-H. Park, M. J. Therien and D. A. Bonnell, ACS Nano, 2010, 4, 1019–1025.
[6] H. Nakanishi, K. J. M. Bishop, B. Kowalczyk, A. Nitzan, E. A. Weiss, K. V. Tretiakov, M. M. Apodaca, R. Klajn, J. F. Stoddart and B. A. Grzybowski, Nature, 2009, 460, 371–375.
[7] M. A. Mangold, C. Weiss, M. Calame and A. W. Holleitner, Appl. Phys. Lett., 2009, 94, 161104.
[8] M. A. Mangold, M. Calame, M. Mayor and A. W. Holleitner, J. Am. Chem. Soc., 2011, 133, 12185–12191.